Biology, Geology and Environmental Science Emeritus Faculty
Image
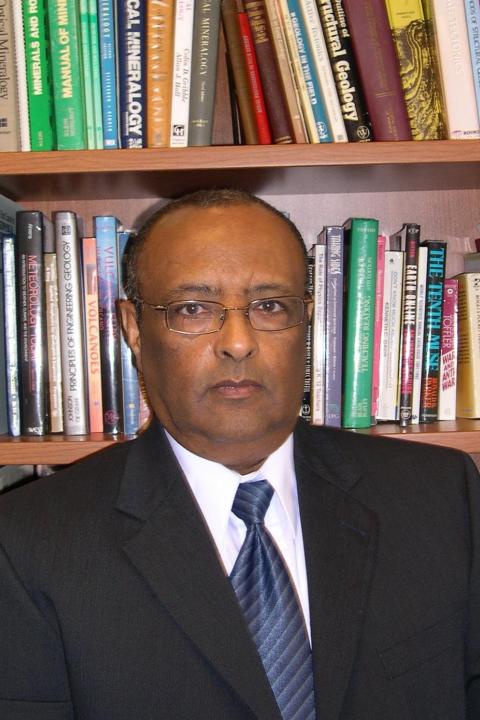
Image
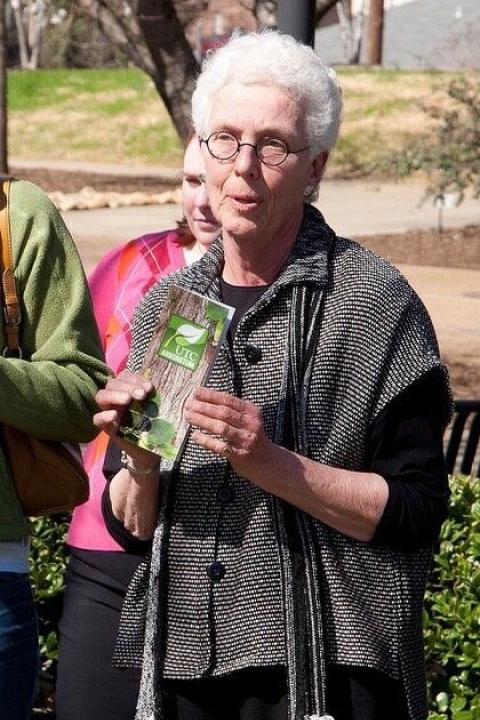
Linda T. Collins
Routt Distinguished Teaching Associate Professor Emerita
Margaret Kovach
PhD
UC Foundation Professor Emerita
Image
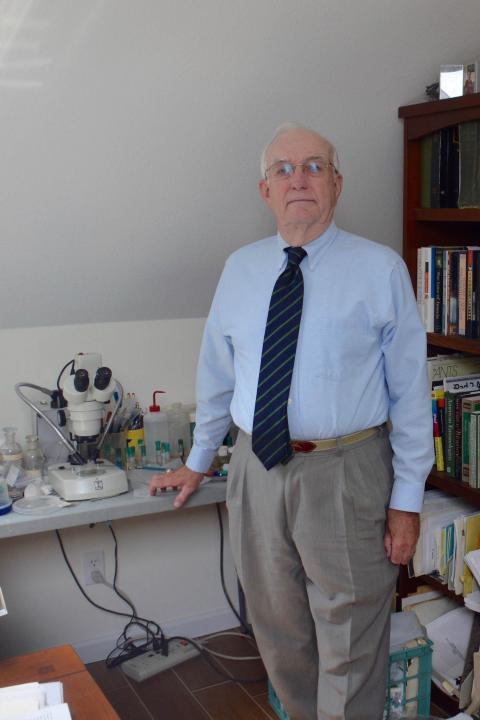
Charles Nelson
PhD
Emeritus Professor
Image
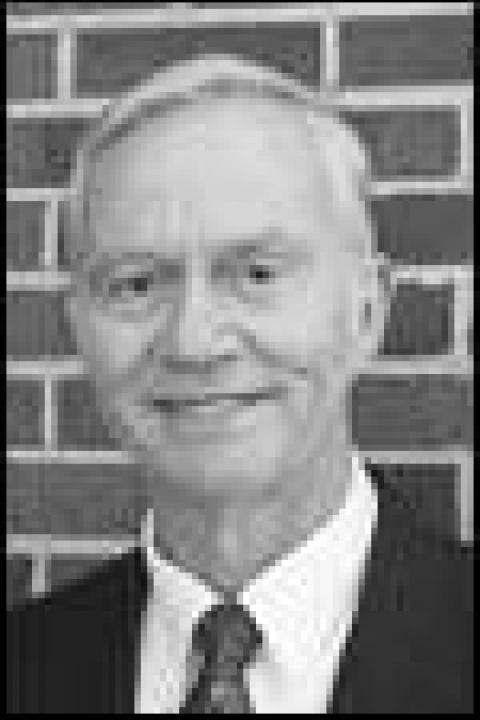
Gene Van Horn
PhD
Professor Emeritus
Jonathan Mies
PhD
UC Foundation R. L. Wilson Professor Emeritus